xxxxxx xx
xxxxxxxxxx
Analysis
NDT
NDT
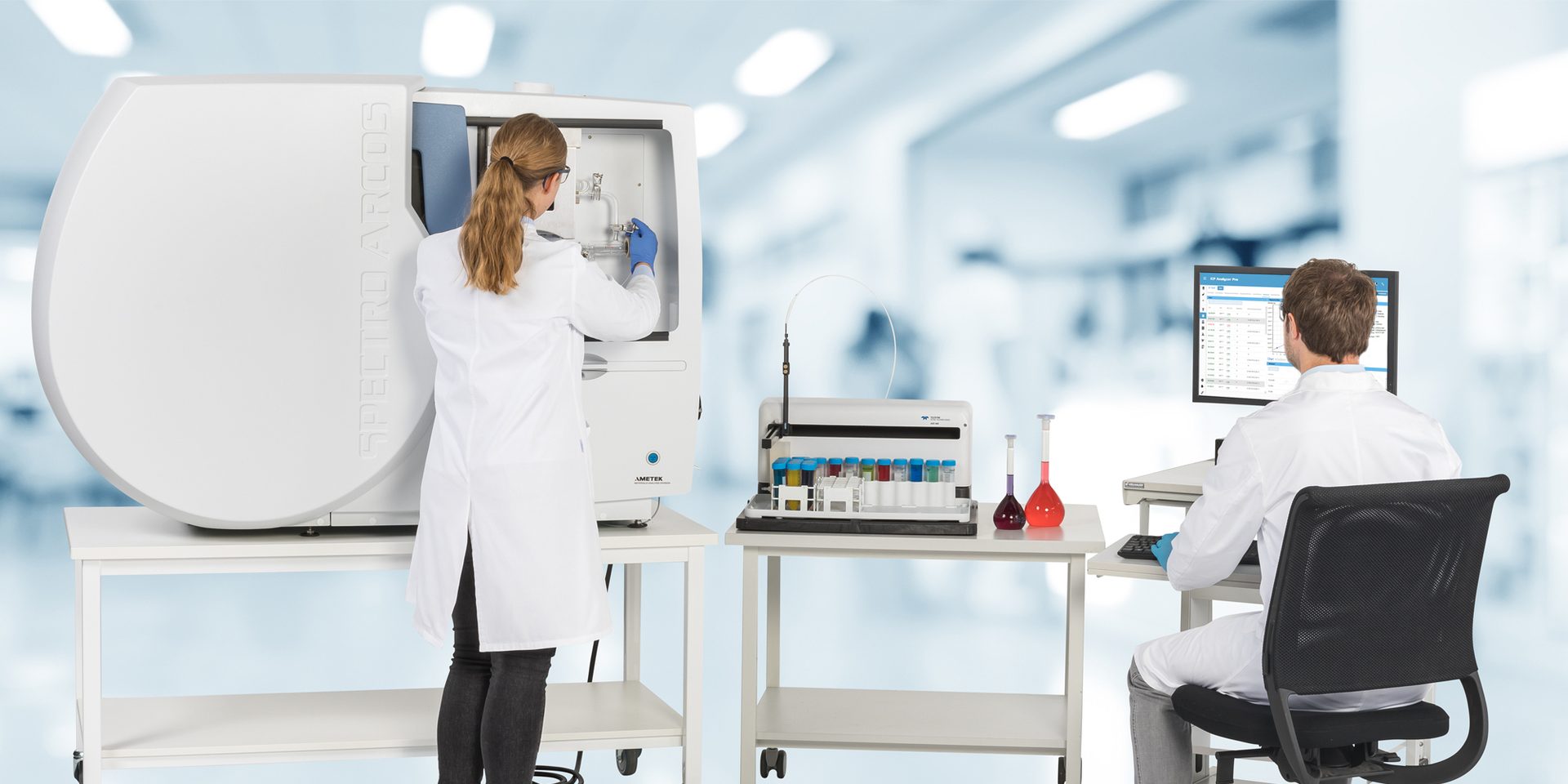
Compositional analysis forms an indispensable building block within additive manufacturing. By Dirk Wissmann
Ensuring Quality Control in Additive Manufacturing Using
Advanced Elemental Analysis
Additive manufacturing (AM), widely recognized as 3D printing, has become a fundamental element of industrial production. It makes it possible to manufacture very complicated parts with quite high accuracy and minimal material losses. While the prospects of using this technology are enormous, quality control of feedstock raw materials and finished goods will be highly relevant for its successful use, particularly in the case of metal parts. The paper addresses the importance of compositional analysis in additive manufacturing, the challenges involved, and the different ways of maintaining stringent quality control.
Additive Manufacturing: Evolution and Challenges
Traditional production processes are revolutionized by AM. Instead of using conventional methods like investment casting or subtractive manufacturing, which creates lots of waste, the parts are built in AM by layer, thus optimizing the materials. In such a process, a possibility of achieving geometrical complexities, lightweight structures, and rapid prototyping is given. But above these listed benefits, one has to deal with challenges, especially for maintaining uniform quality, most especially in metallic materials.
One of the strongest suits of AM is to do with tailoring designs for specific applications in industries as aerospace, medical devices, and automotive manufacturing. At the same time, however, the geometric complexities, with precision required, turn the necessity for strict compositional standards of the materials into a must. Deviation in the elemental composition of feedstock might result in the defects of the final product, to worse performance, durability, or even its safety.
Compositional Analysis: The Imperative behind Additive Manufacturing
Compositional analysis is vital at many stages of the additive manufacturing process. First of all, the processing commences with raw materials, which in AM means the metallic powders. These have to be within chemical specification so that they behave correctly during printing and contribute to the correct properties of the final component. For example, changes in alloy composition can impact melting behavior in the powder, resulting in anomalies in the final product.
Moreover, since the AM process generally involves recycling and re-use of powders, confirmation that materials reused have not degraded or contaminated is important. This will involve analysis to ensure the blend of reused and virgin powders has met the required chemical specifications.
Compositional analysis has become indispensable according to ISO/ASTM 52907-19 on methods for the characterization of metallic powders in additive manufacturing, and ASTM F3456-22 on guiding the reusing of powders in medical applications. The need for confirmation of purity and composition of powders, with correct materials used and changes in composition by reusing them, needs critical emphasis.
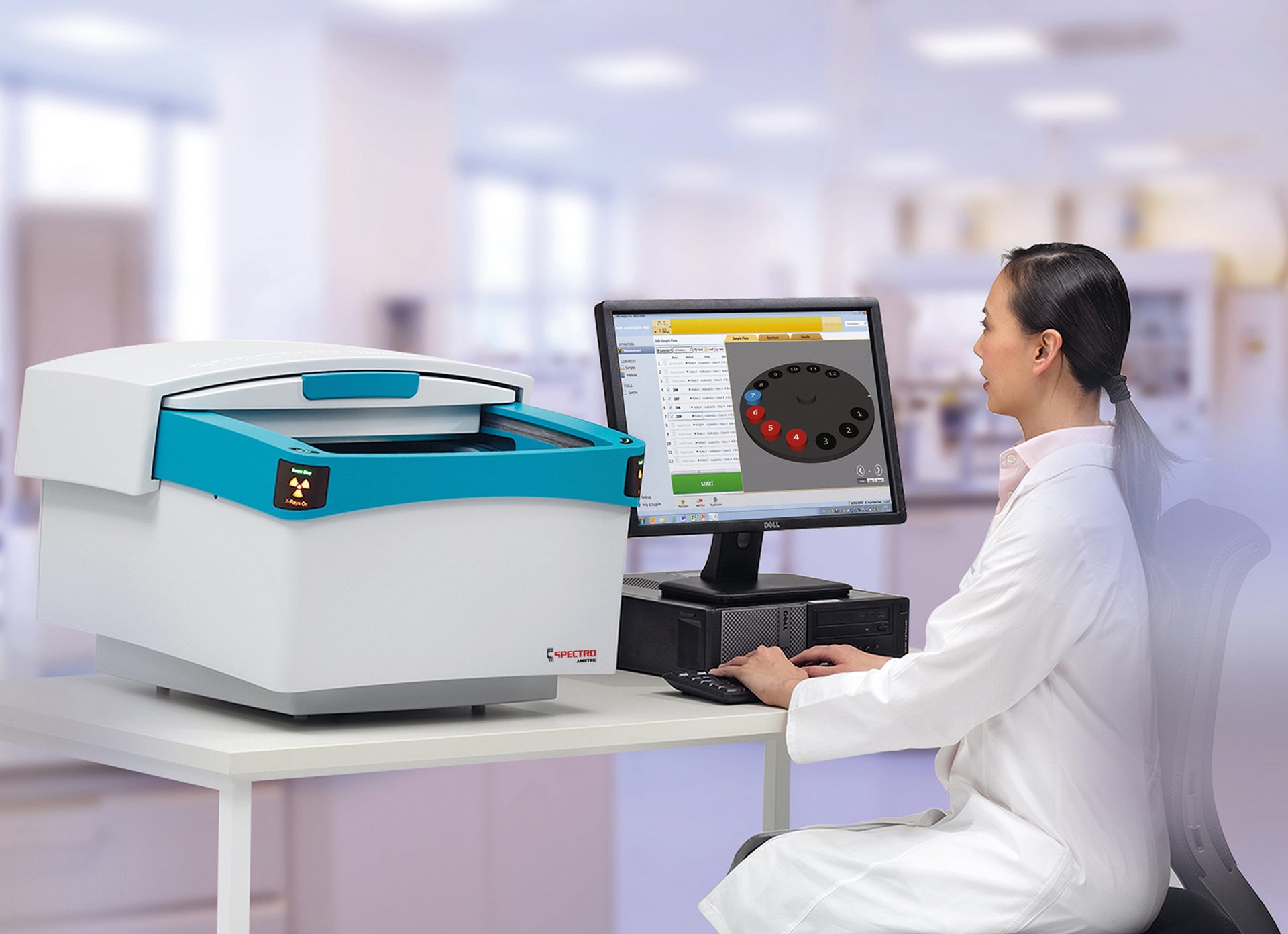
Caption
Techniques for Compositional Analysis
There are several techniques that can be used for a compositional analysis – each has its strengths and weaknesses. Depending on the specific requirements the appropriate technique needs to be selected. For example, the sample material, nondestructive testing or precision are common criteria.
1. Energy-Dispersive X-ray Fluorescence (ED-XRF)
ED-XRF is a nondestructive technique that is particularly suited for the analysis of metallic powders and finished parts in additive manufacturing. It works by irradiating the surface of the sample with X-rays, causing the atoms in the sample to emit fluorescent X-rays at energies characteristic of the elements present. The intensity of these X-rays is measured to determine the concentration of the elements.
One of the major advantages of ED-XRF is the fact that such a wide range of sample types, such as powders, liquids, and solids, can be handled, usually with minimal preparation. This makes it ideal for production monitoring and forensic analyses where unknown materials need to be screened quickly. There are, however, some limitations to EDXRF, including possible interferences from overlapping spectral lines and an inability to detect light elements such as carbon or oxygen.
In consideration of these limitations, ED-XRF is a very useful tool within additive manufacturing, in particular for verification of raw powder composition and homogeneity of blended or reused material. It is also useful for the analysis of finished parts when they fit within the measurement chamber of the instrument.
2. Inductively Coupled Plasma Optical Emission Spectrometry (ICP-OES)
Another analytical technique for compositional analysis is ICP-OES, especially when high sensitivity is a major requirement. It relies on exciting atoms in a sample by means of high-temperature plasma, which will then emit light at a characteristic wavelength after being excited. The resulting intensity from the light emitted by these atoms is measured to quantify the concentration of elements within the sample.
ICP-OES works very well for dissolved samples; hence, this technique is suitable for testing the materials that can be dissolved into a liquid form. It does give very great sensitivity, where it can detect elements in levels below ppm and can handle a wide range of elements across the periodic table. On the other hand, ICP-OES is a destructive technique because the sample has to be dissolved to analyze it, and there is a probability of error during the preparation of the sample.
Within the context of additive manufacturing, ICP-OES finds broad applications in the analysis of metallic powders for elemental composition and trace impurities, which could influence the quality of the final products. Another good application of this technique is the verification of powder composition to ensure the specification requirement by blending powders.
3. Spark Optical Emission Spectrometry (Spark-OES)
Spark OES represents one of the proper and most adequate techniques for the analysis of solid metallic samples, including powders that can be remelted. By initiating a spark between an electrode and the sample, it serves to excite the atoms in the material that consequently emit light. The emitted light is analyzed for the determination of the elemental composition of the sample.
Spark-OES boasts of its analysis speed, whereby a result is normally obtained in less than 30 seconds. This technique is very accurate, obtains a wide range of detection, and also includes elements hardly analyzed by other methods such as hydrogen, oxygen, carbon, and nitrogen. However, Spark-OES requires the sample to be electrically conductive, and the method leaves a small burn mark on the sample. It is not suitable for nondestructive testing of finished parts.
Spark-OES also finds application in additive manufacturing, where it is used to rapidly analyze the composition of solid metal parts against required compositional standards. The technology is especially used to verify the composition of parts remelted from powder due to the fact that Spark-OES can detect even slight deviations from nominal in elemental content.
Challenges in Compositional Analysis
Although the above techniques are highly effective for compositional analysis using additive manufacturing, all of them have their own challenges. For instance, ED-XRF, while nondestructive and easy to operate, may face problems related to light elements and complex matrices; matrix-matched calibrations are required for the highest accuracy. ICP-OES, while highly sensitive, is destructive and very time-consuming since a fully equipped chemical laboratory is needed for sample preparation. While Spark-OES is much quicker, highly accurate, and a more rapid technique, it can only be performed on conductive materials. It also causes a burn mark on the sample.
Other challenges include balancing the speed of analysis with the necessary accuracy or sensitivity to conduct quality control. In numerous manufacturing environments, throughput is a key factor, and sensitivity may need to be sacrificed for speed in certain situations. Applications that have a bearing on critical issues—for instance, in aerospace or medical devices—can always use the finest degree of precision and sensitivity, so lengthier methods such as ICP-OES may be required.
Conclusion
Compositional analysis forms an indispensable building block within additive manufacturing. This technology is under continuous development and is finding its implementation in more and more industries; hence, demands are on the rise for precise, reliable, and efficient quality control methods. Each of these techniques-ED-XRF, ICP-OES, and Spark-OES-offers different advantages; hence, each manufacturer can select a method best adapted to his specific needs.
As such, the use of deployable robust compositional analytical protocols will ensure that the highest quality and safety standards are achieved, thereby creating an avenue for further growth and success of additive manufacturing in the global marketplace. Therefore, the more the industry develops, the more it will rely on improving the development of new analytical techniques and fine-tuning of the existing methods in overcoming the difficulties with quality control, due to which additive manufacture can retain its leading positions in innovative modern productions.